Chirality—derived from the Greek word for “hand”—describes a property that captures one of nature’s most beguiling asymmetries. An object is chiral if it cannot be superimposed on its mirror image, much like your left and right hands. While this might seem like a curious detail of shape or form, chirality is anything but trivial. It lies at the very heart of chemistry, biology, and physics, shaping the structure of molecules, the behavior of subatomic particles, and the very foundations of life.
From the helical twist of DNA to the shape of amino acids and even to the swirling arms of galaxies, chirality manifests at nearly every scale of the universe. And yet, it remains a mystery in many respects—especially in quantum materials, where symmetry governs the behavior of electrons and other fundamental particles. One burning question that physicists have grappled with: can materials that appear achiral spontaneously become chiral under the right quantum conditions?
A Quantum Discovery at Princeton
In a groundbreaking study published in Nature Communications, a team of physicists from Princeton University, led by M. Zahid Hasan, has revealed a hidden chiral quantum state in a topological material that was long assumed to be non-chiral. This discovery not only settles a contentious debate but also opens new avenues for quantum technologies built on symmetry-breaking phenomena.
“We’ve essentially pointed a quantum version of the James Webb Telescope at this material and discovered a previously unseen world,” said Hasan, the Eugene Higgins Professor of Physics. “This is an entirely new class of quantum behavior.”
The Mysterious World of the Kagome Lattice
At the center of this discovery lies a material with a curious geometric structure known as the Kagome lattice—a two-dimensional network of corner-sharing triangles named after a traditional Japanese basket-weaving pattern. It’s a playground for quantum physicists due to its potential to host exotic phenomena such as superconductivity, magnetism, and topological order.
The compound in question, KV₃Sb₅, is one such Kagome lattice-based material. For years, scientists believed this lattice was inherently achiral, its symmetry too perfect to allow handedness to emerge. However, earlier work by Hasan’s group in 2021 hinted at a different story. Using a high-resolution scanning tunneling microscope (STM), they observed a spontaneous charge density wave—a periodic modulation of electronic density—that suggested the possibility of broken symmetry. The findings sparked international intrigue and led to one of the most cited studies in the field.
Spontaneous Symmetry Breaking: A Portal to New Physics
In physics, symmetry breaking is a transformative concept. It’s what turns disordered states into ordered ones—like when water freezes into ice or when atoms align to form a magnet. More profoundly, it explains how the early universe evolved from a symmetric primordial state to the richly structured cosmos we observe today.
In the case of KV₃Sb₅, the emergence of a charge density wave meant that some symmetry had been broken. But which one? Identifying whether chirality was involved required tools that could detect extremely subtle differences between left- and right-handed states—differences that standard instruments couldn’t resolve.
Inventing the Right Tool: The Scanning Photocurrent Microscope
Enter the scanning photocurrent microscope (SPCM), a novel device designed by graduate student Zi-Jia Cheng and postdoctoral researcher Shafayat Hossain. Unlike the STM, which maps the surface electronic states with nanometer precision, the SPCM is tailored to detect optical responses at the quantum level, specifically under the influence of circularly polarized light.
In their experiment, researchers fabricated ultra-clean crystals of KV₃Sb₅, cooled them to just 4 degrees Kelvin (close to absolute zero), and shone circularly polarized laser light onto the sample. At high temperatures, the material was indifferent to whether the light was left-handed or right-handed. But as the temperature dropped and the charge density wave emerged, something extraordinary happened.
“The material started to respond differently depending on the handedness of the light,” explained Cheng. “This is a definitive signature of chirality, known as the circular photogalvanic effect. It proves the presence of broken mirror and inversion symmetry in the charge-ordered phase.”
The Birth of a Chiral Quantum State
What makes this discovery remarkable is not merely the identification of chirality—but the fact that it has spontaneously emerged in a material that is structurally achiral. This is the quantum equivalent of finding a right-handed spiral staircase in a perfectly symmetrical house, built from symmetrical blueprints.
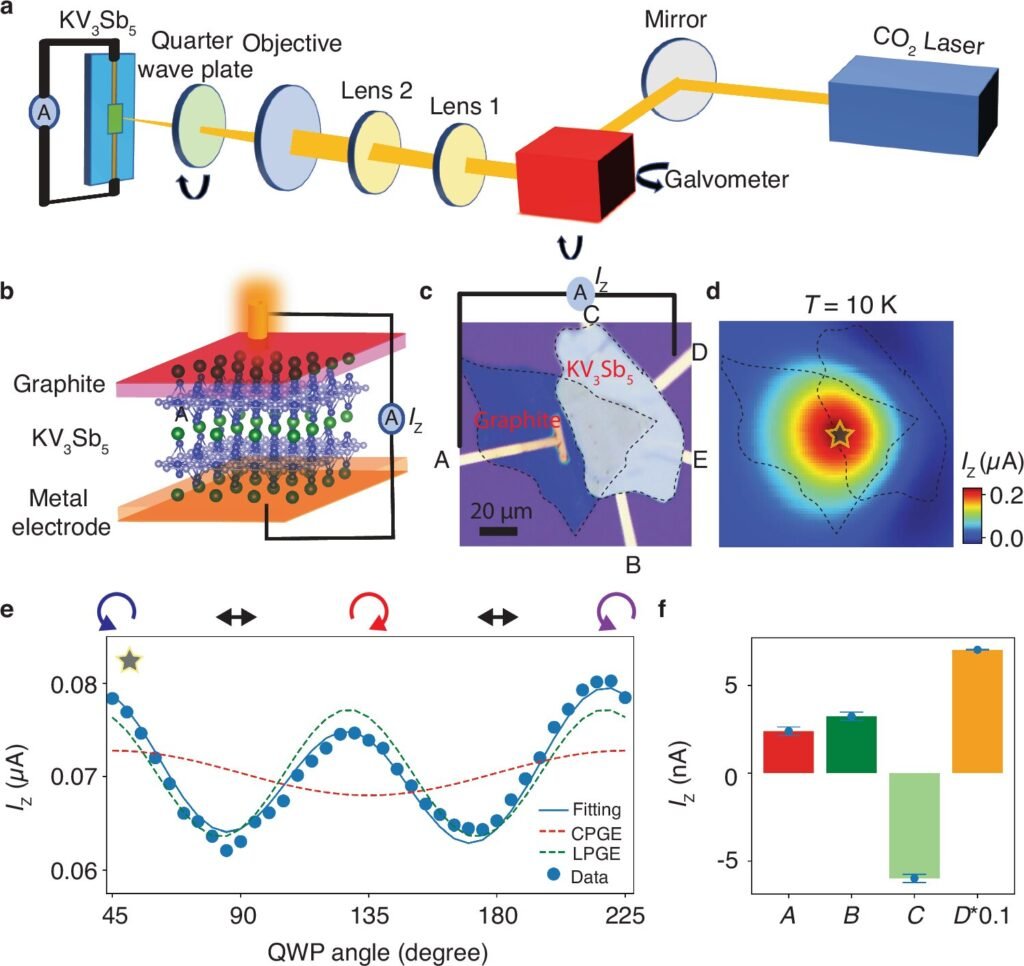
“This is the first time such a chiral quantum state has been observed in a topological Kagome material,” said Hasan. “It’s not just a theoretical possibility—it’s real, it’s measurable, and it’s spectacular.”
The circular photogalvanic effect arises from the asymmetry in how electrons respond to circularly polarized light. The differing photocurrents generated under left- and right-handed light are evidence of a deeper, hidden asymmetry that only emerges under specific quantum conditions.
Unanswered Questions and New Horizons
While the experimental evidence is clear, the theoretical underpinnings are not. “We confirmed the phenomenon, but we don’t yet have a rigorous theory to explain why it happens,” Hasan admitted. “We are staring at something that defies our current models.”
This gap between observation and theory is not a weakness but a strength. It signals that physicists are entering unexplored territory, where new principles may emerge to redefine our understanding of quantum materials. And therein lies the beauty of discovery: each answer leads to deeper questions.
Applications on the Horizon
The implications of this research are vast and could extend beyond academic curiosity. Chiral quantum states are of intense interest for optoelectronics and quantum computing. Their sensitivity to light polarization could be harnessed in next-generation sensors, energy-harvesting devices, and quantum information systems.
Moreover, the experimental techniques developed—especially the SPCM—could become standard tools for probing other mysterious materials. “Second-order electromagnetic measurements, like those enabled by our microscope, are powerful probes for detecting hidden orders in complex systems,” said Hossain.
This work also underscores the importance of interdisciplinary innovation—where ideas from optics, materials science, quantum physics, and nanotechnology converge to unveil new physical realities.
The Deeper Meaning of Symmetry and Its Breakers
Symmetry has always been a guiding principle in physics. The laws of nature, as described by equations, often assume perfect symmetry. Yet the universe, in its grandeur and complexity, is anything but symmetrical. Galaxies rotate, particles spin, and biological systems exhibit unmistakable handedness.
Understanding how and why symmetries break reveals how order arises from chaos, how the universe organizes itself, and how complexity emerges from simplicity. In this sense, the Princeton discovery isn’t just about a strange material on a cold microscope stage—it’s about fundamental truths that echo through every layer of existence.
Looking Ahead: A Quantum Frontier Awaits
“This is just the beginning,” said Hasan, his voice echoing the thrill of exploration. “With these new tools and fresh insights, we’re on the edge of a quantum frontier. Who knows what other hidden phases of matter are waiting to be discovered?”
As researchers continue to dive deeper into the strange and wondrous world of quantum materials, one thing is clear: chirality, that humble twist of handedness, holds secrets far more profound than previously imagined. It not only defines the shape of molecules—it may soon shape the future of technology, theory, and our understanding of the universe itself.
And now, thanks to a brilliant team at Princeton, we’ve taken one more step toward unraveling its mystery.
Reference: Zi-Jia Cheng et al, Broken symmetries associated with a Kagome chiral charge order, Nature Communications (2025). DOI: 10.1038/s41467-025-58262-y
Loved this? Help us spread the word and support independent science! Share now.