In a quiet corner of a University of Chicago lab, beneath the glow of monitors and microscopes, a revolution is brewing—one that begins not in the vastness of space or the crushing gravity of a black hole, but within a single, living cell. Here, hypersensitive quantum sensors—tools forged from the weirdest laws of physics—are being engineered not just to measure time or gravity, but to peer into the very machinery of life.
It sounds like science fiction: Embed a tiny, diamond-based quantum device into a living human cell, track changes in real-time, and uncover the early biochemical whispers of cancer, degenerative disease, or immune dysfunction before they rise to the surface. But a team of researchers at the Pritzker School of Molecular Engineering, in collaboration with the University of Iowa, is turning this dream into something dazzlingly real.
The secret? Not just diamonds—but diamond nanocrystals, engineered like microscopic jewels and polished by the lessons of quantum dot televisions. With a whisper of quantum mechanics and a coat of chemistry inspired by high-definition color displays, they’ve built a new kind of biosensor that thrives in the chaotic, watery, immune-surveilled world inside living cells.
And in doing so, they’ve solved one of quantum sensing’s most persistent mysteries.
The Trouble with Tiny Diamonds
Quantum sensors are among the most sensitive measuring devices known to science. They work by exploiting the fragile, ephemeral states of quantum particles—electrons, atoms, or photons. Among them, nitrogen-vacancy (NV) centers in diamond are a favorite. These are minuscule imperfections in the carbon lattice, where a nitrogen atom replaces a carbon next to an empty space. These flaws, paradoxically, are what make diamond quantum sensors useful. NV centers can be “spun up” or “spun down” and respond to magnetic and electric fields, temperature, and strain with exquisite sensitivity.
In big chunks of diamond, like the kind used in research labs, NV centers can maintain their quantum coherence—the delicate balancing act that keeps their quantum properties alive—for milliseconds, an eternity in quantum time. But these aren’t the diamonds you can implant into a cell.
“Bulk diamond sensors are great,” says Uri Zvi, a Ph.D. candidate at the University of Chicago and lead author of the new study. “But you can’t exactly swallow one. You need something small enough to go inside the cell.”
The solution seems simple: make the diamonds smaller. Shrink them to nanocrystals, just tens of nanometers across, small enough to be ingested by a living cell like a grain of sand swallowed by an oyster. These “nanodiamonds” can, in theory, deliver quantum sensing power directly into the cell’s nucleus, cytoplasm, or membrane.
There’s just one problem. They don’t work.
“When you scale them down, you lose everything that made them useful in the first place,” says Zvi. “The quantum coherence drops. The signal weakens. You’ve got this amazing tool—but in nano form, it’s like it forgets how to do its job.”
This collapse in performance has baffled scientists for years. Why do these nanodiamonds, with the same NV centers as their bulk counterparts, fall apart at the quantum level when made small? Many suspected it had something to do with the surface. Small particles have a lot of surface area relative to their volume, and quantum states are notoriously sensitive to noise—especially from unpaired electrons or dangling bonds at the surface.
So the question became: How do you protect the surface of a diamond nanocrystal without losing its quantum magic?
Lessons from the Living Room
The breakthrough came from an unexpected direction: your television.
Specifically, QLED TVs. These next-generation displays rely on “quantum dots”—tiny semiconductor particles that emit brilliant colors when excited by light. But early versions of quantum dots had a problem. They blinked. That is, their fluorescence turned off unpredictably, making them unreliable.
Researchers fixed this by wrapping the dots in chemically engineered shells. These coatings smoothed the surfaces, suppressed blinking, and stabilized the dots, allowing them to glow consistently and brightly. In a sense, they made quantum dots camera-ready.
Zvi saw a parallel. “What if we could do the same with diamond nanocrystals?” he wondered. “What if the problem isn’t the diamond itself—but what’s on the surface?”
To test the idea, Zvi teamed up with a powerhouse of interdisciplinary talent: Prof. Dmitri Talapin, an expert in quantum dot materials; Prof. Aaron Esser-Kahn, a specialist in immunoengineering; Prof. Peter Maurer, a physicist deeply versed in quantum sensors; and collaborators from the University of Iowa, including theoretical physicists Prof. Michael Flatté and Asst. Prof. Denis Candido.
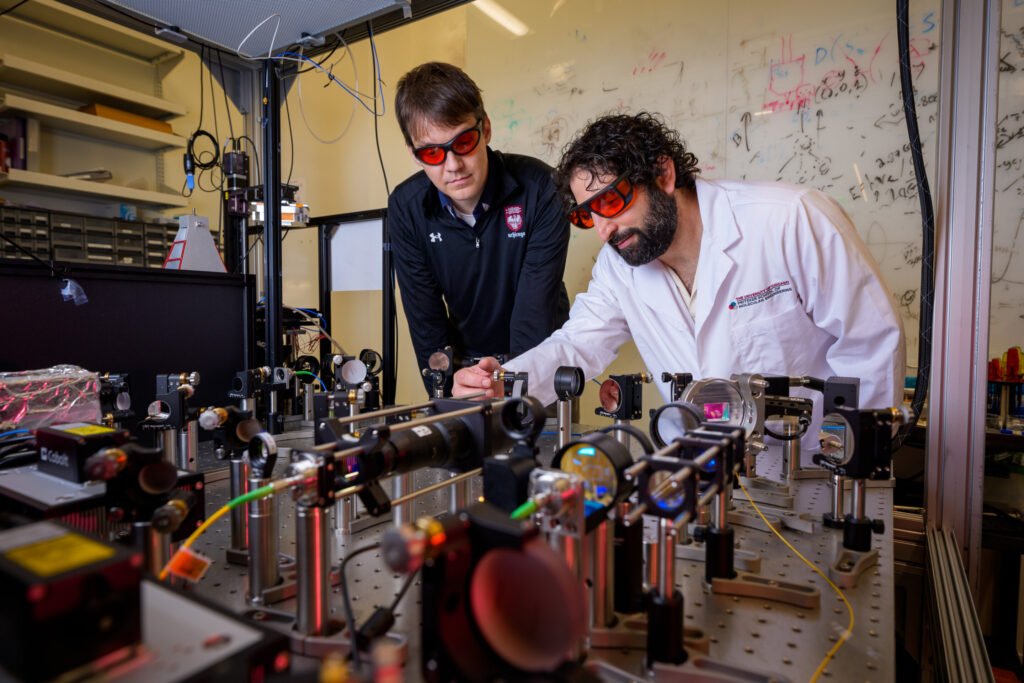
Their goal was audacious: to create a shell for nanodiamonds that would not only protect their quantum properties, but also allow them to sneak inside a living cell undetected by the immune system.
A Shell of Light and Silence
The shell they designed was made of siloxane, a compound of silicon and oxygen with properties that mimic water. Siloxane isn’t just biocompatible—it’s a master of disguise. To the immune system, siloxane looks like a harmless, unremarkable glob of fluid.
“The immune system is really good at spotting what doesn’t belong,” says Esser-Kahn. “But siloxane-coated particles don’t trigger alarms. They get taken up by the cell like candy.”
But would the shell preserve the NV centers’ coherence?
Not only did it preserve it—it enhanced it.
When the team measured the quantum coherence of the siloxane-coated nanodiamonds, they were stunned. The coherence time increased by up to four times. Fluorescence improved nearly twofold. Charge stability—another critical metric—also surged.
“It was shocking,” says Maurer. “We expected maybe a small improvement. What we saw was transformative.”
But it raised a new question. Why was it working so well?
Quantum Mystery Solved
For Candido and Flatté, this was more than just a technical puzzle. It was a doorway into the heart of quantum materials science. Previous theories had focused on the idea that surface defects caused decoherence by generating noise—unpaired electrons that disrupted the fragile NV center states. But that didn’t fully explain the dramatic improvement.
Using sophisticated modeling and quantum mechanical calculations, Candido discovered something new. The siloxane shell wasn’t just passively shielding the diamond—it was actively changing its electronic structure. The interface between the diamond and the siloxane was drawing electrons out of the diamond’s surface, preventing them from forming decoherence-inducing states.
In other words, the shell wasn’t just protecting the quantum signal—it was sculpting it.
“It was a beautiful moment,” says Candido. “We had a fundamental explanation for something that had puzzled the field for years. We were watching quantum behavior being tuned like a musical instrument.”
This insight wasn’t just good science. It opened the door to an entirely new way of thinking about quantum devices: not as static materials, but as modifiable systems whose properties could be engineered through their surfaces.
Into the Cell
With their new quantum sensor in hand—a nanodiamond wrapped in a siloxane shell—the researchers did what no one had done successfully before: they placed it inside a living cell and watched it work.
The results were stunning. The sensor maintained coherence long enough to perform precise measurements of magnetic fields, temperature, and potentially even pH and ion concentration. It could track changes over time, offering a dynamic window into the life of a cell.
Imagine tracking the magnetic signatures of metabolic activity in a cancer cell. Imagine watching, in real-time, how a neuron fires, how an immune cell recognizes a pathogen, or how a stem cell decides to differentiate. With these sensors, that becomes possible.
And because the shell is biocompatible, the cell doesn’t reject it. It doesn’t attack. It doesn’t destroy. Instead, it welcomes the diamond, and the diamond listens.
The Road Ahead
The implications are profound. Medical diagnostics could shift from biopsies and blood draws to internal, real-time monitoring at the cellular level. Early detection of cancer might become as simple as scanning for changes in quantum signals. Drug development could become faster, safer, and more accurate by observing how individual cells respond to treatment. Even neuroscience might be revolutionized by the ability to watch electrical signals in the brain without external electrodes.
And the impact doesn’t stop at medicine. These insights could feed back into quantum computing, materials science, and nanotechnology. The ability to control coherence at the nanoscale through surface engineering is a universal advance, applicable far beyond biosensing.
“This is the kind of science that starts with a problem and ends with a paradigm shift,” says Flatté.
A Symphony of Disciplines
The study—published in Proceedings of the National Academy of Sciences—is a testament to what can happen when chemists, physicists, engineers, and biologists come together. It blends the logic of quantum physics with the messiness of biology, the elegance of material science with the grit of immunology.
And at the heart of it all is a tiny diamond, no bigger than a virus, tuned to hear the silent music of life.
For Uri Zvi, it’s more than a project—it’s a calling. “I didn’t start in quantum science,” he says. “But I found myself drawn into it, because it felt like the most powerful tool we had to answer some of the biggest questions in biology.”
In the end, the work is not just about sensors or signals or shells. It’s about unlocking a new way to understand life—one qubit at a time.
Reference: Uri Zvi et al, Engineering spin coherence in core-shell diamond nanocrystals, Proceedings of the National Academy of Sciences (2025). DOI: 10.1073/pnas.2422542122
Think this is important? Spread the knowledge! Share now.