In the vast and bustling landscape of the developing brain, billions of neurons must undertake epic journeys from where they’re born to where they’ll function for life. These microscopic travelers don’t have maps or highways, yet their ability to reach precise destinations determines everything from how we think and feel to whether our brains function properly at all. Until recently, the idea that neurons might change their “mode of transportation” depending on the terrain they face was more speculative than proven. But a new study has thrown open the door to a deeper understanding of how neurons navigate—and adapt—when the road ahead gets rough.
In a paper published on March 25, 2025, in Cell Reports, a team of international researchers led by Naotaka Nakazawa, a lecturer at Kindai University in Japan, has uncovered a dynamic, mechanoresponsive system that allows neurons to switch between migration strategies based on the physical environment. Their findings are not only fascinating from a basic science perspective—they could also shape the future of brain repair and cancer therapy.
The Intricate Dance of Neural Migration
Neurons are born in specific zones of the developing brain but must travel considerable distances to integrate into neural circuits. Imagine a newborn neuron attempting to weave its way through dense cellular forests, winding corridors, and narrow gaps between brain structures. It’s no small feat. And unlike other cells that might settle where they’re born, neurons often migrate in waves, navigating using internal machinery finely tuned to their environment.
Scientists have long known that different types of neurons use different mechanisms to move. For example, forebrain interneurons use a strategy in which myosin motors push the cell nucleus forward, like a piston in an engine. Meanwhile, cerebellar granule neurons (key players in motor coordination) typically pull their nucleus forward using a different mechanism, relying on actomyosin contractility at the cell’s leading edge.
But what happens when a neuron that usually cruises along a smooth surface suddenly encounters a tight squeeze—a crevice barely wide enough to fit its nucleus?
When the Going Gets Tight, Neurons Switch Gears
Nakazawa and colleagues suspected that migration strategies weren’t rigid. Instead, they hypothesized that neurons might switch mechanisms based on mechanical cues from their environment. To test this, they focused on cerebellar granule neurons, studying how they moved in both 2D and 3D settings.
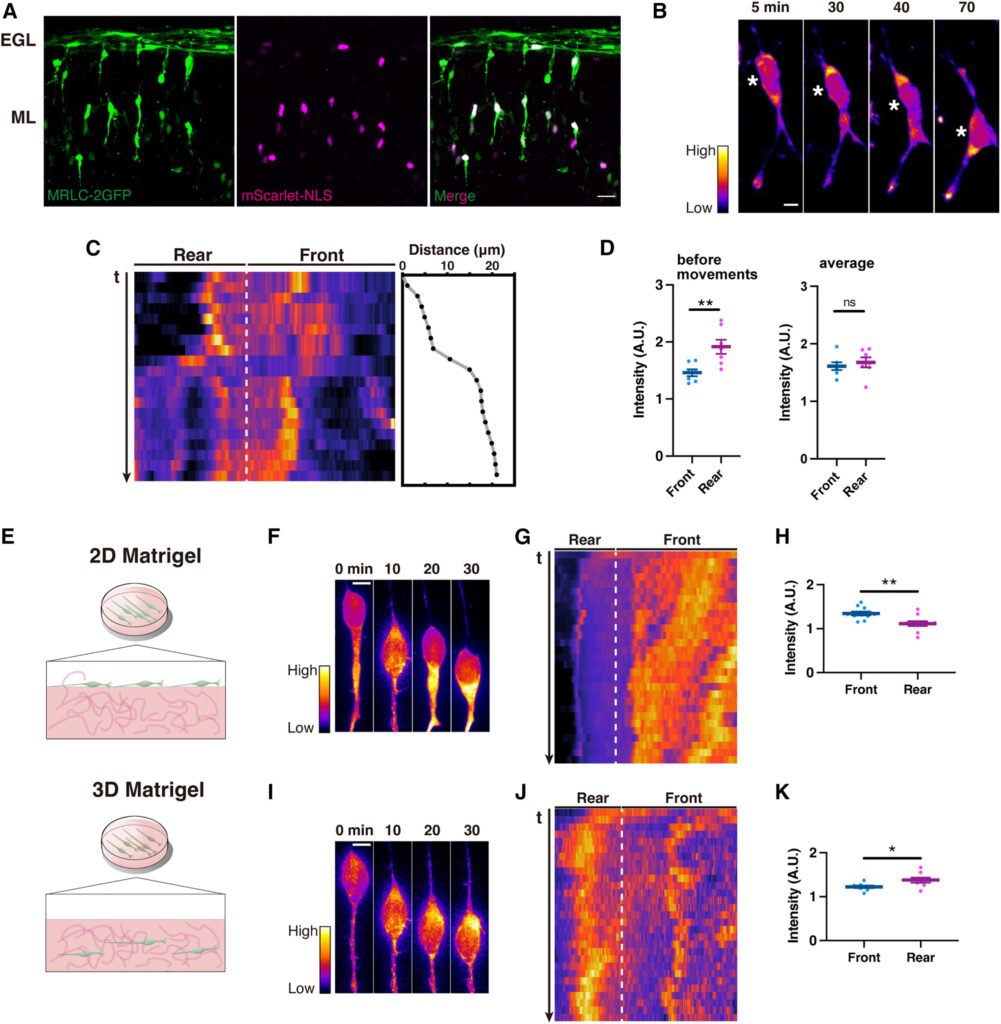
In flat, unconfined 2D environments (like those found in standard cell cultures), the neurons moved using well-documented strategies. They concentrated actomyosin proteins at the front of the cell, generating a pulling force to move the nucleus forward—a relatively straightforward locomotion strategy when space isn’t an issue.
But things got interesting when the researchers introduced microfluidic devices—tiny environments designed to mimic the narrow, confined channels found in brain tissue. These 3D conditions required neurons to squeeze their relatively stiff, bulky nucleus through tight spaces. And that’s where the magic happened: the neurons flipped their script.
Instead of pulling from the front, they repositioned their actomyosin machinery to the back of the cell. This rear-located actomyosin complex generated a pushing force, effectively squeezing the nucleus forward like toothpaste from a tube.
This switch isn’t just clever—it’s essential. Without the ability to adapt, the neurons couldn’t make progress through narrow corridors. And behind this adaptability lies a fascinating mechanosensitive protein: PIEZO1.
PIEZO1: The Neuron’s Built-In Terrain Sensor
The key to this biological versatility is PIEZO1, a mechanosensitive ion channel embedded in the neuron’s membrane. PIEZO1 functions like a pressure sensor. When a neuron enters a tight space and experiences physical stress—like the deformation of its nucleus—PIEZO1 detects that compression and kicks into action.
Upon activation, PIEZO1 opens to allow calcium ions to flow into the cell, setting off a cascade of signaling events. This cascade instructs the neuron to redistribute its contractile machinery from the front to the rear, enabling the shift from a “pulling” to a “pushing” migration strategy.
To prove this mechanism, the researchers genetically disrupted PIEZO1 in cerebellar neurons. The results were striking: in unconfined 2D settings, the modified neurons migrated normally. But when placed in confined microenvironments, they struggled to move at all—confirming that PIEZO1 is essential for adapting to mechanical stress.
Why It Matters: From Brain Development to Brain Repair
This discovery fundamentally changes how scientists think about neuronal migration. Previously, researchers believed that each neuron type employed a fixed migration mechanism. This new study reveals a different truth: neurons are far more adaptable than we imagined, capable of reprogramming their locomotion based on physical cues.
Such plasticity isn’t just a neat trick. It’s likely essential for neurons navigating the three-dimensional labyrinth of the brain, where pathways can vary wildly in width, resistance, and composition.
Even more compelling are the potential medical applications. After brain injury, certain neuroblasts (precursors to neurons) migrate toward the damaged area in an attempt to repair the tissue. If we can better understand how to enhance or guide this migration—especially through the confined terrain of the brain—new therapeutic strategies could emerge to boost brain regeneration.
“Restoring brain function after injury requires getting the right cells to the right place,” says Nakazawa. “Our study suggests that manipulating mechanosensitive signaling might one day help make that happen.”
Beyond the Brain: A Universal Navigation Strategy?
The implications of this work extend beyond neuroscience. Cell migration is a universal process, critical in everything from embryonic development to immune responses to cancer metastasis.
Take cancer, for example. Metastatic tumor cells must travel through dense tissues and extracellular matrices to spread throughout the body. The fact that neurons and cancer cells may use similar adaptive strategies to migrate through confined environments opens new doors for understanding—and potentially targeting—metastasis.
By studying how cells like neurons sense and respond to their physical environment, researchers could uncover universal rules of cell movement, offering new angles for diagnosing or treating a variety of conditions, including:
- Neurodevelopmental disorders, where improper neuronal migration leads to structural brain abnormalities.
- Brain tumors, which may hijack neuronal migration pathways.
- Autoimmune diseases, where immune cells invade the brain or other tissues.
- Wound healing, where skin and connective tissue cells must move into damaged areas.
The Road Ahead: Mapping Cellular Intelligence
What this research ultimately highlights is that cells are not passive entities—they’re problem-solvers. Equipped with molecular sensors and mechanical feedback loops, neurons can assess their environment and choose the best tool for the job.
Nakazawa and his colleagues have now laid the foundation for a deeper exploration into this cellular “decision-making.” Future studies could investigate:
- How different neuron types across brain regions fine-tune their mechanosensing strategies.
- Whether PIEZO1 works alone or in concert with other signaling molecules.
- How manipulating these systems might aid in brain repair or cancer treatment.
As tools like live-cell imaging, organoids, and microfluidic systems become increasingly sophisticated, scientists are poised to uncover the real-time choreography of cell migration with unprecedented clarity.
Conclusion: A Symphony of Motion in the Brain
The human brain is often described as the most complex object in the known universe. What makes it even more astounding is that its architecture is assembled in large part by self-navigating cells that “feel” their way to the right place. Thanks to this new research, we now know that neurons aren’t locked into a single method of travel. They’re equipped with molecular compasses, traction gears, and pressure sensors—and when the path tightens, they adapt and push forward.
It’s a story of cellular resilience, intelligence, and cooperation—an elegant biological symphony that unfolds billions of times over in the developing brain.
And now, at long last, we’re starting to understand the notes.
Reference: Naotaka Nakazawa et al, PIEZO1-dependent mode switch of neuronal migration in heterogeneous microenvironments in the developing brain, Cell Reports (2025). DOI: 10.1016/j.celrep.2025.115405